Cell type: Excitatory Neurons
Visualizing Neurite Outgrowth by Lentiviral Transduction of Fluorescent Proteins into Human iPSC-derived Excitatory Neurons
INTRODUCTION
Elixirgen Scientific’s proprietary transcription factor-based technology allows rapid and reproducible differentiation of human induced pluripotent stem (iPS) or embryonic stem (ES) cells into neurons without sacrificing the purity of the cells1. Our excitatory neurons exhibit typical neuronal morphology with outgrowing neurites and express markers characteristic of a variety of neuronal subtypes, including the pan-neuronal marker tubulin beta 3 class III (TUBB3), the glutamatergic neuron marker vesicular glutamate transporter 1 (vGLUT1), and the cholinergic neuron marker choline acetyltransferase (ChAT).
Lentiviral delivery of a fluorescent protein reporter is an easy, non-invasive and robust method to visualize the morphology of living cells. In this technical note, we describe how our human iPSC-derived excitatory neurons can be efficiently transduced by green fluorescent protein (GFP) reporter lentivirus. Nearly 100% of neurons can be labeled at an optimal multiplicity of infection (MOI) and then analyzed by live-cell imaging-based fluorescence intensity measurement to evaluate neurite outgrowth, test the neurotoxicity of chemical compounds, and to perform various functional assays.
RESULTS
Determining Optimal MOI
Human iPSC-derived excitatory neurons were transduced 4 days post-thaw using a commercial ready-to-use lentiviral vector that harbors GFP under the human synapsin1 (SYN1) promoter. The SYN1 promoter is neuron-specific and therefore is useful to distinguish healthy developing neurons from other cell-types and dead cell debris (Figure 1). Neurites could be visualized 6-7 days post-thaw, and GFP expression was detected in most of the cells on day 8 post-thaw, indicating that these cells were efficiently committed to the neuronal lineage. More intense fluorescence was observed when a higher MOI was used for lentiviral transduction (Figure 2A). GFP intensity continued to increase over several days (Figure 2B), which could be due to both the increasing activity of the SYN1 promoter with neuronal maturation as well as the rapid outgrowth of labeled neurites. Overall, in our culture conditions, a MOI from 2.5 to 10 appeared enough to allow clear visualization of thin neurites. Although we did not notice any significant toxicity at higher MOIs, damaged neurites, namely neurite beading, were apparent upon close observation at MOI 20 (Figure 2C). Due to the risk of cell damage and the cost of lentiviruses, the MOI should be kept as low as possible.

Figure 1. Representative phase contrast and fluorescence microscopy images of Quick-Neuron™ Excitatory - Human iPSC-derived neurons at day 21 post-thaw (17 days after transduction with a GFP reporter lentivirus at MOI 2.5). Hoechst 33342 staining marks nuclei of both live and dead cells whereas green fluorescence shows only live and fully differentiated neurons since GFP is expressed under the SYN1 promoter (scale bars = 200 μm). The differential staining pattern is apparent in the merged image.
Neurotoxicity Studies
Live-cell imaging of neurite outgrowth can be used for evaluating neurotoxicity. For example, treatment with glutamate is known to induce neuronal damage by over-stimulating N-methyl-D-aspartate (NMDA) receptors, a process referred to as excitotoxicity. Using our GFP-labeled excitatory neurons, severe neurite degeneration could be observed with a glutamate concentration of 10 μM (Figure 3). Total fluorescence intensity did not decrease significantly, since most of the cell bodies appeared still intact and retained their membrane integrity at such a low concentration of the compound. Neurite beading is reported as one of the early events of neuronal degeneration prior to induction of cell death, and therefore could potentially be a sensitive assay for early toxicity prediction using high-content screening.
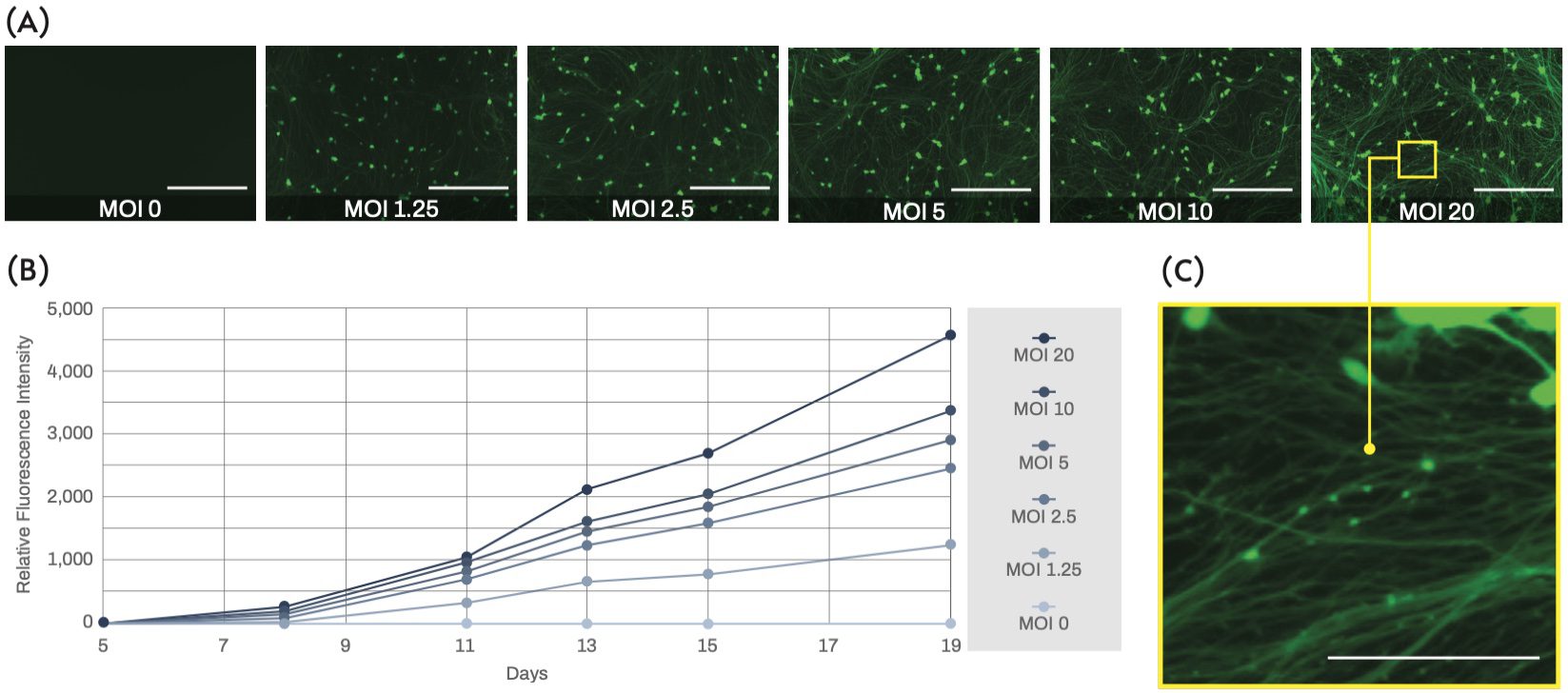
Figure 2. Fluorescence microscopy images of excitatory neurons on day 19 post-thaw (15 days after transduction with a GFP reporter lentivirus) show intensifying fluorescence at higher MOIs (scale bars = 200 μm). (B) The line chart indicates the fluorescence intensity measured in the time-course relative to the intensity measured at day 5 post-thaw as a reference, showing that the signal increases as a function of the MOI and the time after transduction. (C) Magnified view of transduced neurons showing possible neurite damage (beading) at a high MOI of 20.
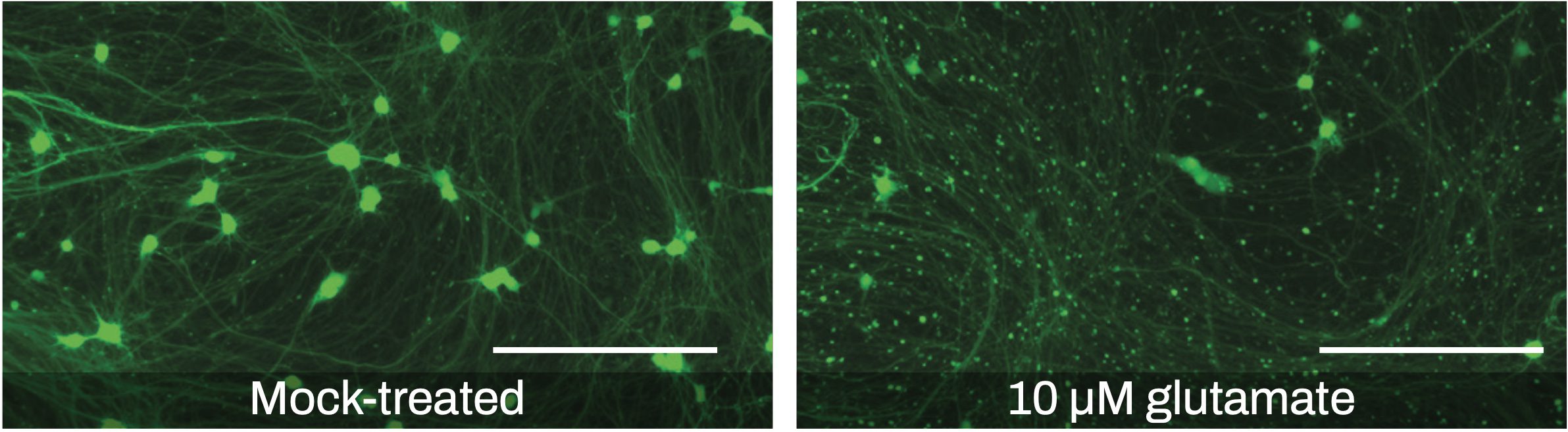
Figure 3. Representative fluorescence microscopy images of excitatory neuron cultures used for the glutamate neurotoxicity assay. Neurons were transduced with a GFP reporter lentivirus on day 4 post-thaw and then treated with 10 μM glutamate for 24 hours on day 21 post-thaw (right). Neurite degeneration or neurite beading induced by excitotoxicity is evident compared to a mock-treated control (left) (scale bars = 200 μm).
Functional Analysis
Lentiviral transduction is also useful for expressing modified proteins for functional analysis of neurons. For example, GCaMP is a genetically encoded calcium indicator based on a fusion of calmodulin, M13 myosin light chain kinase peptide, and GFP, which emits fluorescence when binding to Ca2+. Expressing GCaMP6f (an improved variant of GCaMP)2 in human iPSC-derived excitatory neurons allows neuronal activity to be measured by detecting the intracellular Ca2+ influx evoked by action potentials (Figure 4). Spontaneous calcium oscillations were observed from day 21 post-thaw, which indicates that network maturation induced the synchronization of neuronal firing. The most stable signals, with a regular rate of about 1 peak per minute, were obtained at day 29 post-thaw using a high MOI of 30. The oscillation amplitude was lower than the amplitude observed in standard calcium binding dye assays, possibly because the promoter (CAG) used in this construct was suboptimal for this cell-type. Nevertheless, the lentiviral transduction of GCaMP6f offers the advantage of allowing long-term monitoring of calcium oscillations without significantly affecting cell viability. Changes in neuronal activity can thus be evaluated prior to a more sensitive end-point assay.
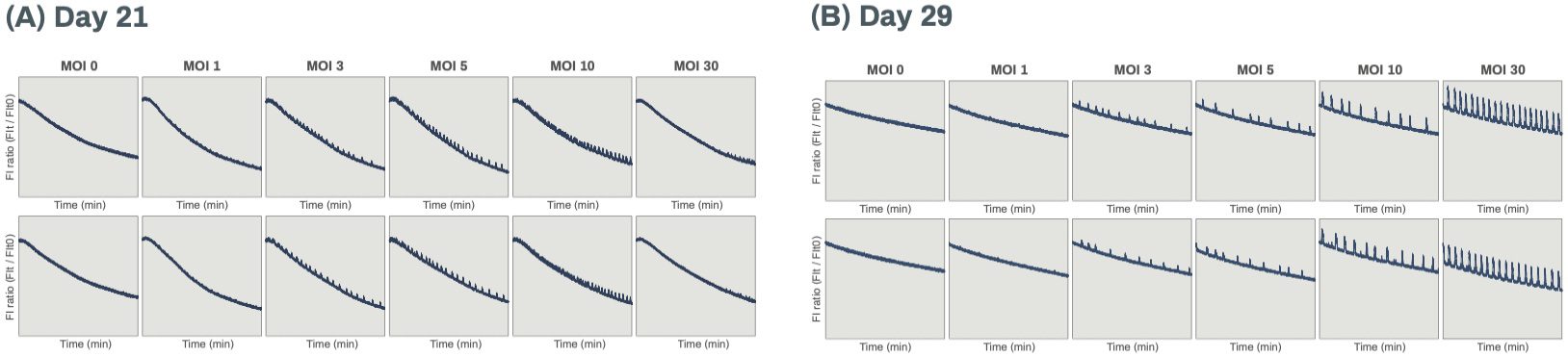
Figure 4. Waveforms of Ca2+ oscillation monitored by GCaMP6f on days 21 (A) and 29 (B) post-thaw in duplicates are shown. Neurons were transduced with lentivirus at the indicated MOIs on day 4 post-thaw. The x-axes indicate the duration of recording (up to 20 min) and the y-axes indicate the intensity of fluorescence detected. The waveforms for MOI 30 in (B) show the most stable signals, with a regular rate of about 1 peak per minute.
DISCUSSION: ADVANTAGES AND CAVEATS
Lentiviral transduction of a fluorescent protein is a convenient method to label cells and has been used extensively to monitor neuronal cells in culture as well as in vivo3. The utilization of neuron-specific promoters, such as the one for SYN1, allows efficient tracking of differentiated neurons4. Our results demonstrate that the majority of viable cells in our excitatory neuron cultures are labeled by GFP expressed under the SYN1 promoter even at low MOIs. This technique allows clearer visualization of neurite outgrowth than common cell membrane dyes such as Calcein AM and DiI, which tend to stain dead-cell debris and/or to be released from stained cells into the medium a few days after staining. In addition, lentiviral vectors permanently integrate into the genome, which assures very stable transgene expression. No decrease of fluorescence has been observed after over 1 month of culture, thereby making lentiviral transduction of GFP an ideal tool for long-term studies.

Figure 5. Fluorescence images of Quick-Neuron™ Excitatory - Human iPSC-derived cells transduced with lentiviral reporter constructs are shown. In these reporters, the expression of GFP is driven by either the CMV (top) or the EF1α (bottom) promoter. The cells were infected at two MOIs indicated above on day 7 post-thaw, and the images were acquired on day 13 post-thaw.
Some caveats of this method should be noted. MOI and infection conditions require optimization for each experimental design, since they can be affected by the cell type, virus lot, timing of infection and culture volume. Selection of the promoter should also be carefully considered depending on the aim of the assay. For example, the human SYN1 promoter is suited to express a fluorescent reporter in a mature neuron-specific manner. On the other hand, the elongation factor-1α (EF1α) promoter is active in a wide variety of cell types, and has been successfully used to label immature neurons. Activity of the CAG promoter in this reporter-neuron combination was not as strong as that of the SYN1 promoter, and a significantly high MOI had to be used to obtain detectable fluorescence (Figure 4). Similar results were obtained with the CMV promoter. We also made minor changes to media formulation because the presence of antibiotics (penicillin-streptomycin) induced cell damage during infection. The use of polybrene and other transduction enhancer reagents is not recommended as they can have detrimental effects on neuronal cell viability5. We found that most of our cells died when some of these reagents were used. Finally, the use of recombinant lentiviruses can raise biosafety concerns, although the most recent self-inactivating (SIN) third generation viruses have been considerably improved on this matter6. Relevant regulations and institutional guidelines should be followed when lentiviruses are handled, which generally requires biosafety level 2 (BSL-2) containment. Despite these practical considerations, this Application Note clearly demonstrates that lentiviral-mediated fluorescence labeling of our Quick-Neuron™ cultures is a powerful and useful method for monitoring cultures, evaluating neurotoxicity, and performing functional assays.
METHODS

Figure 6. Schematic showing workflow of the protocol for lentiviral transduction of fluorescent proteins into Quick-Neuron™ Excitatory - Human iPSC-derived Neurons.
Cell Culture
Elixirgen Scientific’s Quick-Neuron™ Excitatory - Human iPSC-derived Neurons (Catalog number: EX-SeV-CW50065) were thawed and plated at a density of 5 × 104 cells/cm2 following the instructions in the user guide. Minor modifications were made in media formulation for optimal transduction and culture maintenance. On day 1 post-thaw, the medium was changed to Neurobasal Plus (Thermo Fisher) including B-27 Plus, CultureOne supplements, and antibiotics (penicillin-streptomycin) as recommended by the manufacturer. Lentiviral transduction was performed on day 4 post-thaw in Neurobasal Plus Medium without antibiotics to avoid cell damage. Cells were then maintained, with optional addition of antibiotics after day 8 post-thaw, with a half medium change every 3-4 days.
Lentiviral transduction
Commercially available pre-packaged lentivirus particles harboring GFP under the SYN1 promoter (SignaGen Laboratories) were thawed on ice and diluted in Neurobasal Plus Medium without antibiotics at 2×104 transduction units/μL. The appropriate volume of viral suspension was added to each culture to obtain desired MOIs (for example, for MOI 10, 8 μl were added to each well of a 96-well plate which had been seeded with 1.6×104 cells/well on D0). Cells were incubated in a 5% CO2 incubator at 37℃ for 24 hours and the medium was then changed to fresh Neurobasal Plus Medium without antibiotics. Lentiviruses harboring GFP under the CMV promoter or the EF1α promoter were made using a Lentiviral High Titer Packaging Mix with pLVSIN (Takara) and used to infect cells in a similar manner.
Cellular imaging and fluorescence measurement
Images were captured in fluorescence and phase contrast using a BZ-X800 microscope (Keyence). For nuclear staining, Hoechst 33342 solution (Therno Fisher) was added at a final concentration of 5 μg/mL and incubated at room temperature for 30 minutes before analysis. Fluorescence intensity was measured with a Cytation 5 plate reader (BioTek).
Excitotoxicity assay
A stock solution of 1 mM L-Glutamic acid monosodium salt hydrate (Fujifilm Wako) was prepared in distilled water and filter-sterilized. The glutamate solution was diluted to 20 μM in pre-warmed medium and added to the cells with a half medium change for a final concentration of 10 μM. Treated cells were observed 24 hours later.
Calcium oscillation measurements
Elixirgen Scientific’s Quick-Neuron™ Excitatory - Human iPSC-derived Neurons were cultured with human primary astrocytes (Thermo Fisher Scientific) and transduced on day 4 post-thaw using a commercially available ready-to-use lentiviral vector that harbors GCaMP6f under the CAG promoter (SignaGen Laboratories). Calcium oscillation was monitored with the FDSS/μCell system (Hamamatsu Photonics K.K.) for 20 minutes on days 21 and 29.
References
- Goparaju, S. K. et al. Rapid differentiation of human pluripotent stem cells into functional neurons by mRNAs encoding transcription factors. Sci. Rep. 7, 1–12 (2017).
- Chen, T. W. et al. Ultrasensitive fluorescent proteins for imaging neuronal activity. Nature 499, 295–300 (2013).
- Parr-Brownlie, L. C. et al. Lentiviral vectors as tools to understand central nervous system biology in mammalian model organisms. Front. Mol. Neurosci. 8, 1–12 (2015).
- Dittgen, T. et al. Lentivirus-based genetic manipulations of cortical neurons and their optical and electrophysiological monitoring in vivo. Proc. Natl. Acad. Sci. U. S. A. 101, 18206–18211 (2004).
- Bao, F. et al. Polybrene induces neural degeneration by bidirectional Ca2+ influx-dependent mitochondrial and ER–mitochondrial dynamics. Cell Death Dis. 966, (2018).
- Pauwels, K. et al. State-of-the-Art Lentiviral Vectors for Research Use: Risk Assessment and Biosafety Recommendations. Curr. Gene Ther. 9, 459–474 (2009).